Solar-to-chemical energy conversion (artificial photosynthesis)
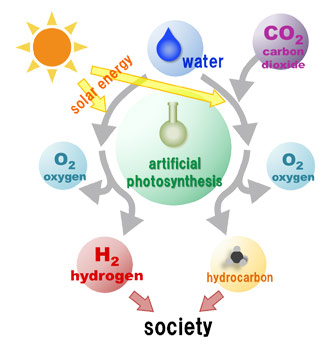
The supply of clean and sustainable energy is regarded as one of the most important scientific and technological challenges in the 21st century. The most probable alternative to fossil fuels is solar energy. Energy harvested from sunlight offers a desirable approach for minimal environmental impact, and what is even better, solar energy is a decentralized and inexhaustible natural resource.
In our laboratory, we are investigating innovative photocatalytic materials for the conversion of solar energy to H2 through a water-splitting reaction and to chemical fuels, such as formic acid, methanol, methane, and hydrocarbons, through CO2 fixation using water as an electron donor. These reactions are classified as “artificial photosynthesis.” Since solar energy mostly comprises visible and infrared lights, we are tackling the development of photocatalytic systems which function efficiently under visible-light irradiation, leading to the realization of sustainable future energy supply.
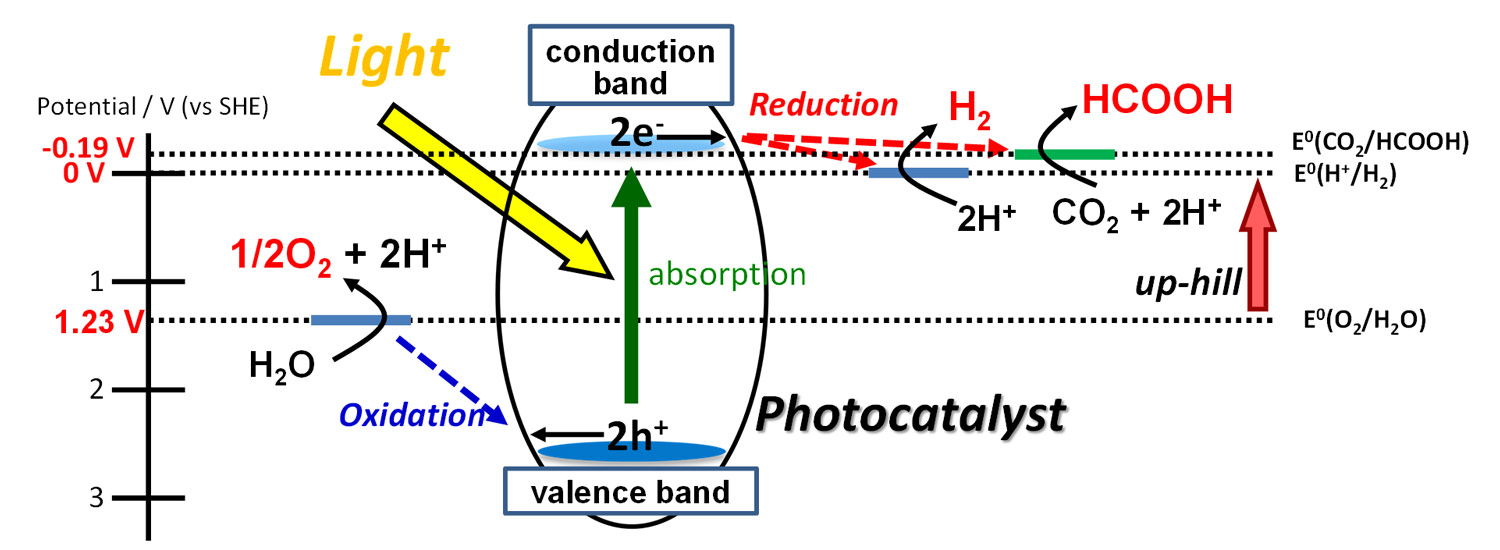
Development of all-solid-state Z-scheme photocatalyst
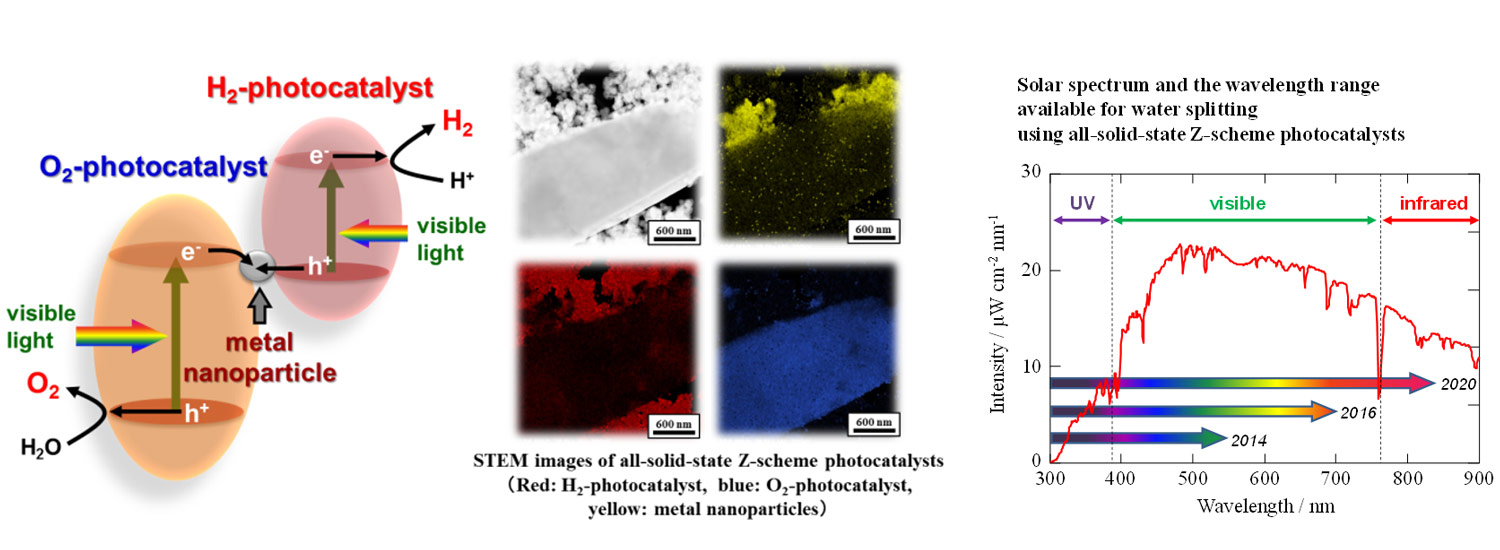
To utilize visible light efficiently, we have developed a novel Z-scheme photocatalyst, so called all-solid-state Z-scheme photocatalyst. This Z-scheme photocatalyst is composed of visible-light-sensitive H2- and O2-photocatalysts combined through metal nanoparticles, and the inserted metal nanoparticles mediated the transfer of photoexcited electrons from the conduction band of the O2-photocatalyst to the valence band of the H2-photocatalyst. Thus, the photoexcited electrons in the H2-photocatalyst and holes in the O2-photocatalyst can effectively reduce and oxidize water, respectively, thereby accomplishing overall splitting of pure water (i.e., distilled water without chemicals) to produce H2 and O2 at a ratio of 2:1. Using this photocatalyst, we have demonstrated overall water splitting up to 850 nm, representing that the infrared light, as well as the entire range of visible light, is available. In addition, we have recently found that this photocatalyst also has the potential to reduce CO2 under visible light.
Design of multi-electron transfer catalyst
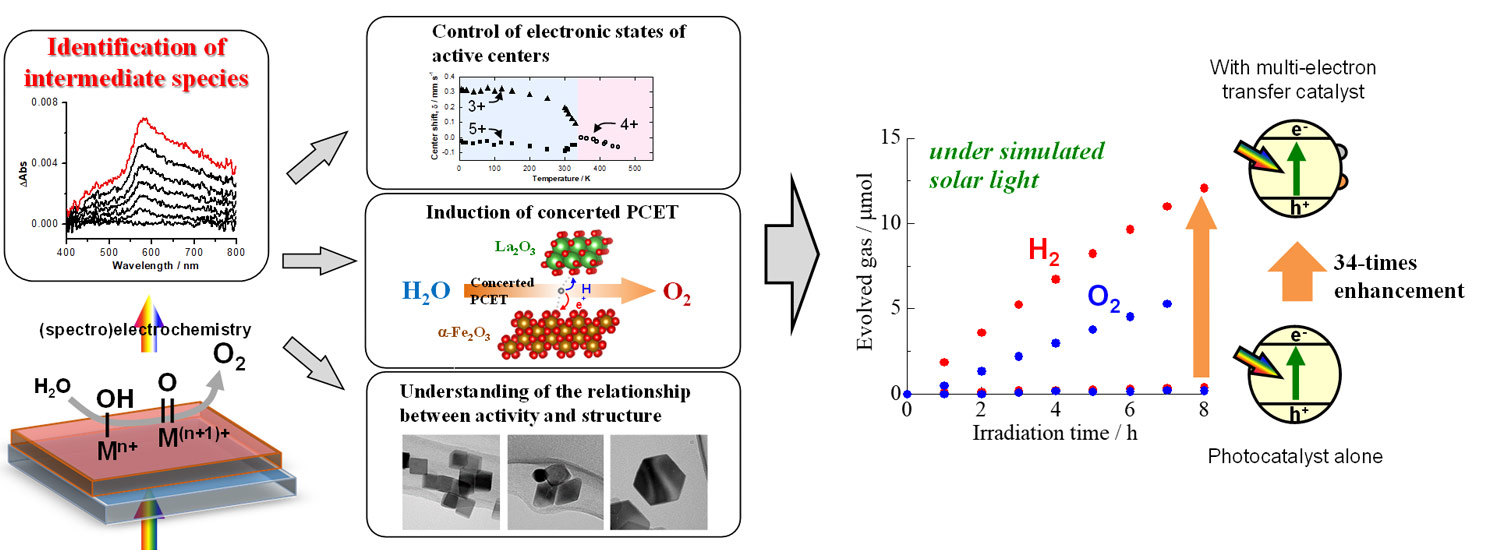
In a multi-electron transfer reaction, multiple electrons transfer to a reaction substrate simultaneously, and this reaction has thermodynamic advantage over a stepwise one-electron transfer reaction. Therefore, the multi-electron transfer reaction is essentially important for various energy conversion processes including artificial photosynthesis, and numerous studies have been conducted to develop active catalysts. However, the underlying principles of how multi-electron transfer reactions proceed remain unsolved. In order to gain insight into the design of efficient multi-electron transfer catalysts, we have identified the limiting factors of multi-electron transfer reactions using (spectro)electrochemical techniques and developed various catalysts based on the findings. Furthermore, thus-obtained multi-electron transfer catalysts are combined with photocatalysts and studied to achieve efficient solar-to-chemical energy conversion.
Thermal, light-to-electric energy conversion
Thermoelectricity is a technology for the direct conversion of heat to electric power. Recently, energy harvesting has been attracting attention to realize the IoT (Internet of things) society. Unused, ambient thermal energy is one of the most important energy sources for energy harvesting. Solar power, including both photon and thermal energies, is also regarded a s one of the most important ambient, renewable energies. We have already demonstrated an enhancement of the thermal-electric conversion efficiency by utilizing the photon energy, accompanied by a photochromic phenomenon. This demonstration can lead to a multifunctional material as a component of photochromic smart windows, which are expected to provide shade from sunlight while simultaneously generating electric power.
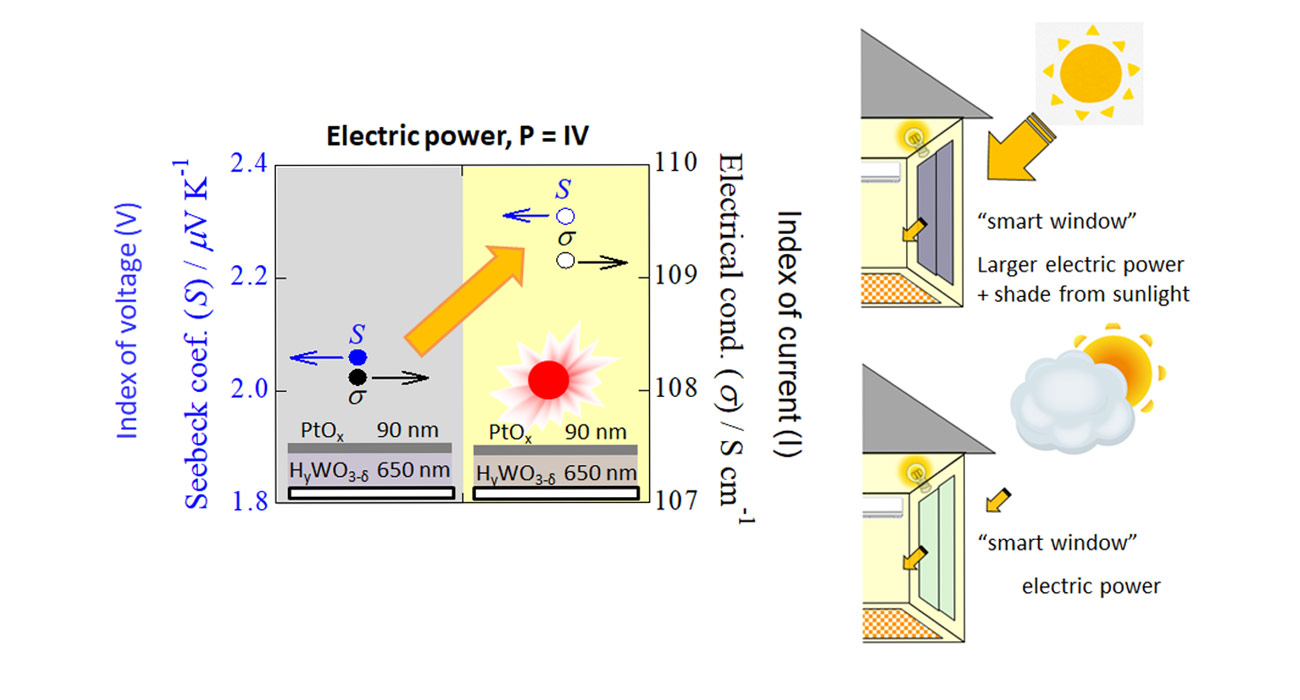